Physiology of Managing T1D
Understanding the physiologic basis of glucose production and utilization is critical to T1D glucose management - Article 3 of 7 in Systems Approach Series
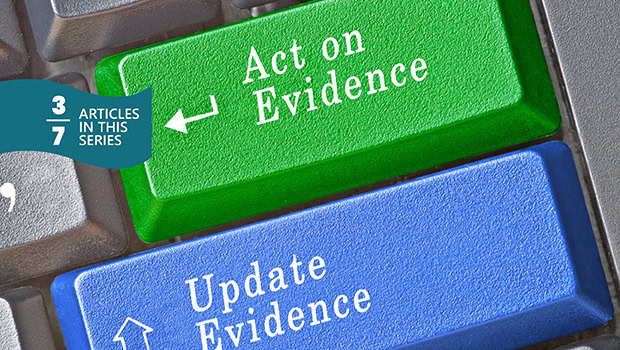
Glucose measurement technology has advanced and this has enabled the control strategies that can be used today.
Introduction
Our body needs the energy to work, and there are just a few ways for cells to get that energy: oxidizing (burning) either fat or a sugar known as glucose. Glucose is the result of the digestion of carbohydrates in the gut (stomach and duodenum) whence it is absorbed by blood and delivered around the body. From the vascular (circulatory) system it infuses interstitial tissue, where (together with circulating oxygen) it provides energy to body tissues.
This glucose concentration for people with T1D can range widely, including (from low to high):
- Too low for the brain to do its work (think, understand and reason) properly
- The normal range for healthy, non-diabetic people
- Higher than that, but still without medical consequences
- So high that spending time at such levels can lead to the diabetes complications of kidney disease, vision loss, erectile dysfunction, neuropathy, stroke, atherosclerosis, heart attack, poor circulation in the feet leading to gangrene, and slow wound healing with its amputation risk.
At the low end, normal fasting glucose ranges from 60 to 99 mg/dl, with pregnant women and children trending lower than others. Testing mental function shows hypoglycemic effects cannot be detected above 55 mg/dl, making 55 to 60 or 70 mg/dl a ‘be alert about trending lower’ range, and 40 or 45 to 55 mg/dl “mild” hypoglycemia. Severe hypoglycemia has been defined as where you can’t remember after recovering and shows up around 25 mg/dl. The high 20s to the low 40s range is not “severe” in that sense, but far from mild. In a hospital setting, where they don’t like to get even close to trouble, they consider 70 mg/dl or below hypoglycemic.
On the high side, after eating, normal people will climb to as much as 140 mg/dl. For diabetics, of course, keeping post-prandial glucose that low is difficult, so for them, the renal threshold of 180 mg/dl (where the kidneys release, or “spill”, glucose into urine) is considered the safe upper limit. Glucose higher than that can result in the consequences listed above over time, based on a combination of the degree above and amount of time spent there. A random glucose test of over 300 mg/dl deserves serious concern, as it reflects a lack of adequate (or any) insulin, in which case the body falls back on using fat, its other source of energy. But burning fat produces ketone bodies or ketones, and since they are acidic, one-way physicians have to identify uncontrolled diabetes is when there is too much of them. This is called diabetic ketoacidosis, which is a combination of ketones and acidic (low pH) blood, and it can ultimately lead to diabetic coma and even death. Beyond this, a random glucose exceeding 500 mg/dl may result in preemptive hospitalization for this very reason.
Glucose from Body Stores
Food is the main thing that leads to a glucose increase, but other things cause it to rise as well.
The liver can both absorb and release glucose. It consumes glucose it absorbs to produce fatty acids that are then used to manage inflammation in the body. In addition, it can produce glucose in two ways: from previously stored glycogen (glycogenolysis) or from precursors such as lactate and glycerol (gluconeogenesis). These sources of glucose are important when animals don’t or can’t eat because unlike muscle and fat cells, glucose is the only source of energy for the brain (nerve) and blood cells.
Glucose produced internally by the liver is particularly important when we don’t eat during sleep. A few hours before waking there is a process that prepares the body so it has the energy needed to begin the day (think breakfast when you have to hunt or gather it). At first growth hormone is released, and that stimulates the liver to produce glucose. That early morning glucose is responsible for the “dawn phenomenon” familiar to Type 1 diabetics, where glucose rises like the sun.
These all raise glucose, but the other factor that affects glucose, lowering it, is insulin. Exercise lowers glucose as well, but that physiologic process still involves insulin. Exercise just affects the body’s sensitivity to insulin and prolongs its effect during recovery from the previous exercise. The healthy pancreas in normal operation is told to begin releasing insulin by the brain roughly the same time it has your salivary glands prepare for the food.
Insulin is produced in the pancreas islets of Langerhans, along with other hormones. The alpha cells produce glucagon, the beta cells produce insulin and amylin, the delta cells produce somatostatin, the epsilon cells produce ghrelin (which regulates appetite), and the gamma cells produce pancreatic polypeptide.
The beta cells work behind the scenes generating insulin which is stored internally until needed. When you eat, insulin circulating in the blood needs to rise rapidly to a useful level, and the beta cell does this by releasing a relatively large amount all at once in a “first phase” of insulin release. Afterward, basically the rest of the time, it produces the “second phase” of insulin release, which is a modest amount that enables circulating glucose to be used as needed, after which the second phase winds down to just a background level. You can think of bolus insulin as a manual first phase and basal insulin as a manual second phase. That’s why the emphasis is placed on the bolus insulin speed of action, with Regular “fast”, rapid-acting, and now extra fast inhaled insulin available in the market. Second phase background insulins include intermediate-acting NPH, and long-acting Detemir and Glargine. Basal insulin itself, however, involves more than just long-acting insulin, since the insulin action needed for basal homeostasis (steady glucose) needs to increase during the dawn phenomenon, and long-acting insulin can’t do that too.
Insulin Administration
The fundamental difference between administering insulin by syringe and insulin pump is that the bolus of insulin that forms a subcutaneous depot with a pump is in just one place, the location of the inset. This is usually moved every two or three days, but for that time all the insulin goes into one depot. This is an issue because the absorption time for a depot is proportional to the surface area of the depot, and the surface to volume ratio changes with size. The surface is proportional to the square of the diameter, while the volume is proportional to its cube. The upshot of this basic physics is that small depots absorb faster than large ones because of the larger surface per unit volume that small depots have.
There is another factor at work as well, one that physicians know and mention all the time: intra-muscular (IM) and subcutaneous (SubQ) injections. The former goes into the muscle (which is used for injecting many infectious drugs), while the later go into fat or fatty tissue. Whether from a pump inset or syringe, all insulin should go SubQ. Even with SubQ, syringes can be different, because the body part used can change. SubQ insulin can be injected in the thighs, lower loins, abdomen, back, and the upper (posterior) or lower (lateral) part of the upper arm. Of them all, the abdomen is the fastest to absorb and is where bolus insulin should be injected for maximum overall speed.
So the best basal insulin administration is with carefully timed pump rates varied over the day, while the best bolus administration is with rotated sites in the abdomen by syringe. And the best administration size is with many small injections rather than a single full sized one. I try not to use more than four or five units for each individual bolus injection. The new 30 unit syringes are great for that. I still draw up however much I want for the bolus, but I inject just the units I want, then remove and re-insert the still full syringe into the next site, repeating until it’s all used up.
Short History of Blood Glucose Measurement
Managing blood glucose has always been difficult, and for years measuring it was impossible outside a laboratory. The alternative at that time used the fact that high BG, above the renal threshold for glucose (approximately 180 mg/dl), caused glucose to “spill” into the urine. In fact, diabetes was first recognized by observing that urine produced in this situation attracted flies. With the time required for blood glucose to move through the bladder into the urine, however, even the presence or lack of urine glucose only showed what blood glucose had (very roughly) been earlier. That lack of a quantitative quality to urine measurements (using Tes-Tape that just indicated presence or absence) contributed to the simplistic control regimen, utilizing nutrition consistent in amount and timing, that existed at the time.
But when I was diagnosed in 1984, user-based (consumer) blood glucose tests had just become available, and my endocrinologist went to some trouble to alert me to Chemstrips. Today, a version of Chemstrips is used for urine testing, but the original product allowed an estimation of blood glucose from a large “hanging drop” of capillary blood taken from a fingertip and placed on the two-part chemical pad where you let it sit for a minute, then wipe it off and wait another minute for the pad colors to develop. The colors were then compared to a set printed on the side of the tube container the strips came in. There were only nine glucose levels to compare, at 20, 40, 80, 120, 180, 240, 300, 400 and 800 mg/dl, each with colors ranging from pale light to quite dark. You could try to interpolate between these color shades if the strip didn’t match perfectly, but back then this was not only good enough but far superior to urine testing. When eventually meters came along to read the chemical pads automatically, they determined a numerical glucose value by measuring their brightness.
Today, by contrast, the FDA doesn’t think most continuous glucose monitors (CGM) are good enough for standalone use, but only as an adjunct, asserting users should perform an independent glucose test (using up a strip costing 30-75 cents) before dosing. Thanks to today’s new technology, their standards have changed since the Tes-Tape and Chemstrip days when accuracy within 20-25% was as good as it got. Dexcom has responded as well, with their latest G5 and G6 CGMs approved by the FDA for “non-adjunctive” dosing, and accurate within about 10%.
While early glucose test strips all measured blood glucose, different brands did it differently. They all used raw capillary blood, which includes both plasma and hematocrit, the red blood cells that result in anemia if too low. The amount of hematocrit can vary, however, making the glucose concentration in blood (the part without blood cells) uncertain when using whole blood if you don’t know its level of hematocrit. Worse, the normal range of hematocrit is different for men and women (with men’s higher), and there is very little overlap between the two. But when researchers studied something with a glucose effect of some sort, they always used a laboratory test that reported the value for just the blood plasma. They did that because hematocrit doesn’t contain glucose and this way they knew the intrinsic glucose concentration, in just the plasma. This made plasma glucose seem “better” somehow.
So one glucose strip maker’s marketing department (I suspect) got the idea to include a filter for the blood so that only the plasma would get through to be tested. This made their strips similar (in that sense) to laboratory testing that measured just plasma glucose, and for a few years there was considerable controversy over capillary vs. plasma glucose readings, because other makers proceeded to produce “plasma equivalent” readings by assuming a standard value of hematocrit (converting the value with a formula), and they didn’t even use one value for men and another for women. Standard strips today all report plasma (equivalent) values, but the old filter-based version that started it all probably cost more and is unavailable today, so there is no uncertainty about capillary vs. plasma glucose any more, but by using a formula the absolute accuracy of the “plasma equivalent” value is still not the same as you’d get from a lab testing just the plasma itself.
Blood glucose values are a transitory thing in any event, and physicians naturally want an idea of their patients’ glucose control with some sort of overall measure. Fortunately for them, glucose becomes attached to hemoglobin over time, a process called glycosylation, and the measure of this, abbreviated HbA1c, is proportional to the glucose the hemoglobin has been exposed to. Hemoglobin itself lives for roughly 120 days, so HbA1c reflects 120 days of glucose, more or less. The newest red blood cells, however, only reflect a few days’ glucose and will have little glycosylation, while the oldest will incorporate everything for almost four months as well.
This glucose exposure of hemoglobin over anywhere from 1 to 120 days for each daily cohort makes HbA1c a weighted average of glucose over four months, not one of the better-known averages such as the mean, median or mode. You will see folk refer to A1c as a “two to three-month” average glucose level, but that’s a simplistic view that ignores the last month entirely, and fudges the middle two. It’s really this triangular weighted average. The weighting is roughly 40% for the most recent 30 day month, 30% for the month before that, 20% for the 30 days three months ago, and 10% for the oldest 30 days from three to four months ago.
Short History of Insulin and Insulin Dosing, including Pumps
Historically, Type 1 diabetes was incurable and eventually led to death, with the only effective treatment a no-carbohydrate diet that was impossible to maintain. In 1921 Frederic Banting and John Macleod, with the help of Charles Best, investigated the pancreatic ducts of healthy dogs and in December 1921 obtained from their islets of Langerhans a saline extract that kept a severely diabetic dog alive. This was announced at an American Physiological Society conference at Yale University on December 30, 1921. During the next year, together with James Collip, they managed to purify the extract with alcohol to precipitate the active ingredient they called “insulin”, based on the islets (islands) they came from, announcing it at the Association of American Physicians on May 3, 1922. Banting and Macleod received the 1923 Nobel Prize in Physiology or Medicine for this discovery. By the end of 1923, insulin had been in commercial production for a year by Eli Lilly and Company in Indianapolis, and with it, diabetics recovered from comas resumed eating carbohydrates (in moderation), and had been given a new lease on life.
At first industrial insulin production came from either slaughtered beef or pig pancreas extract. Insulin derived from pigs was closer to human insulin, differing in just one amino acid, while beef differs in two. But while “porcine” was more like human, religious folk who don’t eat pork didn’t want to use their insulin, either, and lived with beef.
In part, for this reason, there was always a desire in the pharmaceutical industry to produce an insulin identical to human without, of course, using human pancreas extract. That finally became possible when recombinant DNA technology was developed in the 1970s. Genentech, an early leader in this technology, managed to use information from a human gene to have yeast synthesize an effectively human gene product. With their new technology, they first produced somatostatin in 1977, and then synthetic human insulin in 1978. When this human insulin was first produced commercially, the manufacturer (Eli Lilly) promoted it heavily to the market that had been using beef.
The first human insulin worked faster than porcine, and much faster than beef, and the users at the time had some trouble getting accustomed to it because they had no direct way to see how it worked over time. They could only see how it worked on them, typically when used the same way they used their prior insulin.
Fortunately for me, I came down with T1D a few years after human insulin became available and never used animal insulin, though I was limited to Regular and NPH insulin until Lispro and Aspart were developed in the mid-1990s [see the 1st article, My T1D journey].
As mentioned in [1st section, my T1D journey], most medical treatment in the 1980s was based on a simplistic feedback-control understanding used to adapt to the necessarily consistent diet. Patients settled on a diet they could live with, with a regimen of two shots a day, morning and evening, each consisting of mixed NPH and Regular insulin. This was known as the split-mixed regimen, and the dose quantities for the four components was adjusted until glucose was neither too high nor low for as much of the day and night as users were comfortable testing.
In the years before NPH insulin and the split-mixed regimen, glucose control used (animal) Regular insulin with presence and absence of urine glucose the measurement means. This required at least four shots a day, which motivated the split-mixed regimen after NPH came along, but from an engineering control perspective, despite the extra injections, that original protocol was probably at least slightly better. In particular, when I first started evaluating the state of the art, I wondered how NPH could be simultaneously good for lunch (from the morning NPH shot) and overnight (from the evening shot). One covered a meal, after all, and the other background insulin needs. In particular, there was a graph of insulin action for the split-mixed regimen that had a hump for the Regular and another for the NPH. The two humps looked good, but I realized the total insulin action was their sum, which was never plotted. I thought it suitable for a book “How to lie with graphs”, to go with an existing title: “How to Lie with Statistics”, by Darrell Huff, published in 1954.
I mentioned this to a highly respected diabetologist, Dr. Julio Santiago, and he immediately phoned someone at Eli Lilly. Years later I noticed that a sum total plot had been added in some of their material, but sadly, Dr. Santiago had died by then.
By the 1980s, when I became T1D, glucose testing had recently become possible, and I quickly gravitated to the basal-bolus regimen, both for its flexibility in meal amount and timing and for the improved control it enabled. It may be implemented with both CSII (Continuous Subcutaneous Insulin Infusion) and MDI (Multiple Daily Injections), though CSII allows better tailoring of the basal dose than does MDI, because it used Fast acting Regular insulin at first, and after the mid 1990s, Lispro and Aspart, with the insulin activity tailored for every hour or two of the day by the pump.
So technical improvements with serendipitous timing provided the rapid-acting insulins suitable for the basal-bolus regimen just as the BG testing it required became available. Insulin pumps were greatly improved since their development, as well. The first CSII unit was too big to carry, requiring a backpack, but in 1976 a wearable version was devised that formed the basis for a company called “AutoSyringe”, which Baxter Health Care bought in 1981.
In addition to the basal dose timing flexibility noted above, pumps basically force the use of a basal-bolus regimen, making it impossible to use the NPH of a split-mixed regimen. Wearing a pump means you always have insulin at hand, making bolus use convenient as well as flexible. Today’s pumps have microcomputer smarts built in, as well, providing for a record of infusion history, and the ability to have the pump suggests an appropriate bolus dose using not just meal content, but insulin on board and current BG. Basal and bolus infusion may be implemented within tiny dose increments, as well, without the dose dilution recommended for fractional units manually.
Of course, it’s not entirely good, either. Pumps not only cost thousands of times more than syringes, but their infusion sets only last a few days, making for an ongoing expense. The infusion sites each get far more insulin than with syringes that have their sites rotated, making for a hard bump under the skin called lipohypertrophy. Over time these can make it hard to find a suitable site for the infusion set cannula, which affects insulin absorption relative to a fresh site. The infusion set adhesive can contribute to an allergic reaction, as well. Wearing one all the time can preclude swimming and rough sports, not to speak of the discomfort. Should it fail, a pump replacement isn’t as handy as a syringe, though manufacturers try to replace them within days.
After 20 years of MDI I opted for an insulin pump with my Kaiser health plan (with a co-pay much higher than for their drugs), but these disadvantages, especially the discomfort of having to wear it in bed overnight, bothered me. I found a two-pocket stretchy wrap product made for the purpose to let me wear it in bed, which helped, but after about two years I decided to gave up on my pump and go back to using MDI.
Abbreviations and References
(see Master List)
amylin |
|
Blood Glucose (aka BG) |
|
blood plasma |
|
C-Peptide |
|
capillary |
|
CGM |
|
cohort |
|
CSII |
|
dawn phenomenon |
|
duodenum |
|
FDA |
|
gangrene |
|
ghrelin |
|
glucagon |
|
glucagon |
|
gluconeogenesis |
|
glycerol |
|
glycogenolysis |
|
glycosylation |
|
growth hormone |
|
hematocrit |
|
I.M. injection |
|
interstitial |
|
islets of Langerhans |
|
ketoacidosis |
|
lactate |
|
lipohypertrophy |
|
Multiple Daily injections (aka MDI) |
|
pancreatic polypeptide |
|
renal threshold |
|
somatostatin |
|
Split-Mixed |
https://medical-dictionary.thefreedictionary.com/split-mixed+insulin+dosing |
SubQ injection |
|
vascular system |
Content of Series
There are six articles in the series plus a dedicated section on Acronyms and References.
- My T1D Journey as a Systems Engineer
- Physiology of Managing T1D
- Food Effect on Glucose
- Basal Insulin Management in T1D
- Bolus Insulin Management in T1D
- Using CGMs, Insulin Pens, and Pumps
- Acronyms and References
See description of full article series here.